Lab rotation: Ocean Chemistry
Lab leaders: Shannon Burns & Juan Millan-Otoya
Peer counselor: Shiyenne Muentes
Lab observers/assistants: Naja Murphy & Delfina Navarro-Estrada
Written by, Shannon Burns, OCG Fellow
This year’s Ocean Chemistry lab made for some good reactions! We began with a bead counting activity to show the composition of salts in the oceans. Campers quickly learned that minor chemical species make up only ~0.7% of sea salts, and in that category is iron (Fe). Although Fe has a low concentration in seawater, it’s required by phytoplankton (microscopic marine algae at the base of the food web) to grow, and therefore is considered a micronutrient (nutrient needed in low concentrations). The supply of Fe to phytoplankton is super important to us humans too, since globally phytoplankton provide about half the oxygen in our atmosphere as they grow (photosynthesize)!
Like people, phytoplankton are selective about their food. What type of Fe do phytoplankton prefer? Dissolved Fe! Ironically though, Fe doesn’t stay dissolved well in the oceans. That’s where dissolved siderophores come in, produced by marine bacteria. A siderophore is a type of ligand (molecule that wraps around a metal atom and captures it) that binds (sticks) preferentially to Fe. Siderophores steal the Fe atoms from particles (like dust) in the water, dissolving the Fe as a result. Phytoplankton then steal the dissolved Fe from the siderophores. Through a Plinko board activity representing these concepts, campers learned that phytoplankton are creative chemical foragers who rely on both bacteria and ligands to grow.
To visualize all this steeling in real time, we did an experiment. When Fe in steel wool interacts with oxygen, rust forms. Campers placed steel wool balls in solutions of water and tea. They found that the water ball rusted overnight, but the tea ball didn’t, and the tea had turned from brown to black. Why was that, when both solutions contained oxygen? Well, the tea had Fe-binding ligands called tannins that stole Fe from the wool and dissolved it in the tea, turning it black! Without that reactive Fe, the wool couldn’t rust. Thus, campers could see the power of ligands to dissolve Fe.
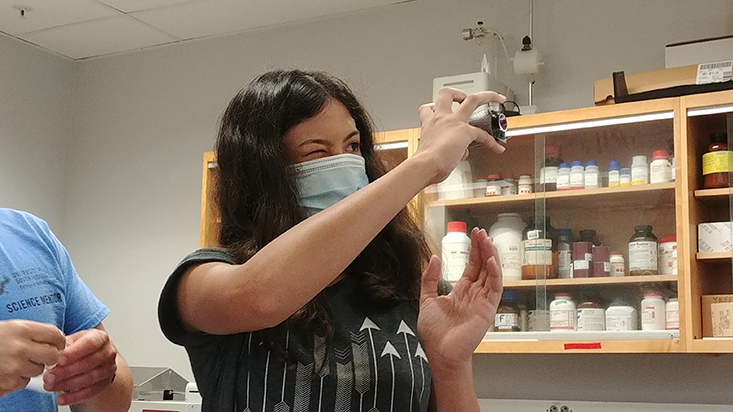
Camper Sydney checking that the pH cell windows are clean and clear for light to pass through once in the spectrophotometer.
Other chemistry can also affect phytoplankton survival in the oceans, such as the pH of the water (how acidic or basic it is), and the water’s ability to resist changes in pH (alkalinity). Since seawater has a bicarbonate (HCO3-)/carbonate (CO32-) buffer system, it can resist becoming more acidic when carbon dioxide (CO2) from the atmosphere dissolves into the ocean. Campers dissolved dry ice (pure CO2) into tap water colored with indicator dye (which changes color with changes in pH) to visualize how CO2 input makes tap water more acidic. They then competed in respiration races using seawater and tap water colored with indicator dye to show how the seawater was slower to change in pH when campers exhaled CO2 from their breath into the water, thanks to its buffer system. The camper who managed to changed the pH the most in each race found it pretty breathtaking!
Unfortunately, the ocean’s buffer capacity cannot keep up with rapidly increasing CO2 concentrations in the atmosphere, causing ocean acidification. Ocean acidification negatively impacts organisms that use calcium carbonate (CaCO3) in their skeletons (ex: shells, corals, and coccolithophores, a type of phytoplankton). At higher pH, CO32- is more available. Lower pH means less CO32-, making it difficult to form CaCO3. Campers visualized this by dissolving a shell in hydrochloric acid during the lab. They were quite shell-shocked by the reaction.
The final section of our lab highlighted the need for precision and accuracy when collecting data. Put simply, stay on target (accurate) with consistency (precision)! Campers compared the methods they used on their research cruise with the methods oceanographers use in the lab to measure the salinity (saltiness) and alkalinity of water samples collected on the R/V Angari. They also compared different methods of measuring pH, and learned about the importance of using certified reference materials (CRMs) to identify the accuracy of a sample run. They concluded that our lab’s methods were more accurate and precise than those used on cruise.
It was so pHun to give these Females a crash course in marine chemistry! My favorite part about this lab is having watched it evolve over the years to give more visibility to the normally invisible parts of the ocean, often for the first time in campers’ lives. I’ll never forget the bonds we all made this year. :)
View the slideshow below.